This article was originally published in the International Journal on Tall Buildings and Urban Habitat Issue II, April 2018
Abstract The 435-meter Akhmat Tower in Grozny, Chechnya, Russia, will be shaped to refer to the Nakh tower, a traditional watchtower typology in the region. A four-sided, pyramidal shape interacts gracefully with an eight-sided geometry at the base. The mixed program, complex geometry, and high seismic and wind conditions of the region demand a sophisticated design response, particularly in terms of the façade and the structural engineering, in order to achieve a coherent, crystalline form.
Design Concept In 2014, Adrian Smith + Gordon Gill Architecture (AS+GG) was commissioned to design a signature tower in Grozny, Chechnya, a Republic of Russia located in the North Caucasus region, near the Caspian Sea. The vision for the 435-meter Akhmat Tower is based on one of Chechnya’s most symbolic architectural typologies: the Nakh tower (see Figure 1).
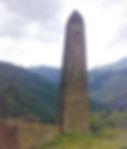
The design of the new building shares a strong geometric relationship to this traditional watchtower in its architectural proportion, the use of a four-sided pyramidal shape, and a monumental top. Four sloping walls taper to reach the observation and museum floors at the top of the building, taking advantage of an intriguing crystalline volume, which features views of the presidential palace, the Akhmad Kadyrov Mosque, and the distant mountains. The eight-sided geometry of the lobby and ground plan, formed by two intersecting squares, also has a strong cultural reference to traditional geometric patterns found in the region (see Figure 2).
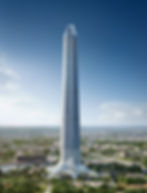
Tower Program Akhmat Tower has an approximate gross area of the program is organized in order to utilize ideal lease spans in each zone. The 250,000 square meters, comprising a variety of programmatic elements. Because the building tapers, building has one basement level, with parking and mechanical spaces. The main lobbies are located on Levels 1 and 3, with visual access to the eight-story atrium above. Each program type has separate drop-offs, including hotel, residential, office, and observation, on different sides of the building. Retail is incorporated with the observation drop-off. The hotel ballrooms, wedding suite, grand lobby, and restaurants are all located at the upper (Level 3) lobby.
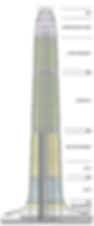
Within the tower, the office program occupies the lower 10 levels of the building, followed by seven hotel guest room floors, and 11 levels of serviced apartments. The upper 49 levels of the tower are residential units, culminating in two levels of penthouse units at the top. The pinnacle features a signature restaurant, an observatory, and exhibit space (see Figure 3).
Structural Approach to Extreme Seismicity and Wind Conditions
The key drivers of the structural system selection for Akhmat Tower were the extreme seismicity and wind conditions of the region. For example, seismic design parameters for this site exceed those utilized for design in San Francisco by 50%. Seismic demands of this magnitude, for a tower of this extreme height, require unique structural systems with extreme ductility and stiffness. Conventional tall building structural systems, such as a reinforced-concrete core-and-outrigger system, simply do not have the ductility required. Local wind speeds utilized for design and service ability of the tower were equivalent to those recorded in coastal regions of the world.
The structural system designed and customized for Akhmat Tower is an evolution of the exterior perimeter tube, utilized on iconic tall buildings such as the Willis Tower in Chicago. The lateral system is concentrated on the exterior of the tower and is comprised of 4.5-meter-spaced, high-strength columns that are connected through a network of highly ductile one-meter-deep perimeter beams. Once constructed, this ductile-tube building system will comprise the world’s tallest special moment-frame seismic-resisting system. To optimize the structural system’s material requirements and minimize the seismic forces on the tower, high-strength steel with a minimum yield strength of 690 MPa (S690QL) was utilized for the vertical column elements in the special moment frame. A highly ductile steel material, with a minimum yield strength of 390 MPa (C390), is utilized for the special moment-frame coupling beams. These beam elements also use reduced beam section (RBS) flange reductions, in accordance with the provisions set forth in the American Institute of Steel Construction (AISC)’s Seismic Design Manual. Ductility was further improved at these locations by flaring the top and bottom flanges between the RBS locations and the faces of columns.
Internationally recognized performance-based design (PBD) approaches were utilized for the evaluation of both seismic and wind-induced responses, considering the less-frequent life-safety design events and the common higher-frequency serviceability events used to evaluate reparability, drift and occupancy comfort.
The seismic design performance requirements considered site-specific hazard analyses conducted by experts in both the US and the Russian Federation. These regional evaluations were utilized to develop site-specific synthetic ground motion records for evaluation and optimization of the tower structural system. Life-safety maximum considered event (MCE) ground motions represented a 2,500-year return period earthquake. Different motion characteristics were utilized to evaluate the structural member strength and ductility in several cases, in order to prevent collapse. More frequently-occurring seismic event records were similarly developed to evaluate tower drift and reparable damage.
With the extreme wind climate in Grozny, a detailed and exhaustive wind engineering and wind tunnel testing program was conducted by RWDI, in Guelph, Ontario, Canada. Three different wind tunnel test model techniques were utilized to evaluate the wind loads and tower responses; High Frequency Force Balance (HFFB), High Frequency Pressure Integration (HFPI), and an Aeroelastic test, which allows for direct measurement of the additional aeroelastic damping inherent in the structure’s inertial response. In addition, a detailed evaluation of the local wind climate, based on airport and government wind station and balloon data, was conducted.
Auxiliary Damping Strategy
As the extreme seismicity of the site required a very stiff and robust structural system to resist the seismic design forces, resistance to high wind-induced design forces was manageable. However, while it was necessary to minimize the tower weight to keep the seismic inertial forces in check, the light tower system led to wind-response challenges for serviceability, particularly in the case of accelerations at the top of the tower associated with occupancy comfort. In order to mitigate these accelerations, two compound opposed-pendulum tuned mass dampers (TMDs) will be installed at the tower top. These passive damping devices will provide inertial resistance to tower motions that are developed under service level wind speeds, reducing one-year return period tower accelerations to eight millig-units, which is below internationally accepted perception limits,making the Akhmat Tower very comfortable for its occupants.
The two TMDs are located on Level 96 – above the residential floors and directly below the observation floor (see Figures 4 and 5). Because of the tapering shape of the tower, floors become quite small near the top of the building, and finding enough space for the TMD was a significant challenge. The layout of the core required that the express and fire-fighting elevators be located at the center of the building,necessitating a non-centralized location for the damper in plan. Additionally, the building maintenance machines needed to coexist with the damper on the double height MEP floor.
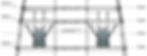
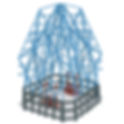
It was decided early in the design process that the damper would be split up into two locations on each side of the core. Although two dampers are not as space-efficient as a single damper overall, it was found that the two dampers did sufficiently reduce the tower accelerations, as previously noted, which made it a feasible approach.
In addition to the challenges of fitting the dampers in plan, the height requirement of the damper in order to achieve the necessary swing of the cables presented another challenge. The solution was to allow the cables to extend up through floor 98 – into the observation deck. Glass handrails will be built around the cables, and a glass floor will be installed, so that visitors to the observation deck can view the TMD. In this way, the TMD will be used as an architectural feature and an educational exhibit, showing building visitors how dampers are used in supertall buildings.
The creation of the accordion-shaped exterior wall (see Figure 6) in Revit was a complex process, which was done using Rhino, various plug-ins, and translated to Revit using adaptive components. The typical exterior wall family was a panel that contained 12 main geometry points. The most complex part of the exterior wall modeling process was to build the tapering corners and the trapezoidal panels that flare and taper as they connect the main exterior wall to the atrium. In order to build such a complex wall natively in Revit, the use of adaptive components was critical in order to document all of the different panel types in the tapering base of the building. One advantage of the design was the symmetry– as it was only necessary to build one eighth of the main exterior wall, which could then be mirrored eight times.

Performative Massing and Exterior Envelope Design
The overall form of the building was influenced by consideration for wind performance combined with enhancements to the user experience and aesthetic of the tower. Early on in design, it was determined that the overall tapering pyramidal shape was intrinsic to the contextual design concept and building function. However, it was also noted by the design team that a square-shaped plan with a flat top and sharp,square corners is not an ideal wind performance profile for a supertall tower –thus, design refinements were made with improved wind performance in mind.
An initial force-balance test of an early conceptual design, which included a completely flat top and square corners,yielded a wind-load response that was estimated to be up to 15% greater than the final design, which featured the 2-meter chamfered corners and a 45-meter-tall,sculpted, crystalline top (see Figure 7). The design process for the top of the building was thus influenced both by the wind tunnel results, and by an aesthetic desire for a more crystalline form, which worked together in influencing the final design. The shaping of the bottom of the building also showed a measurable benefit in pedestrian wind comfort versus an extruded building base, as it was found through wind test data that pedestrian wind comfort in the spring,summer and fall would generally be in an acceptable range without mitigation, and measures to improve wind comfort in winter (such as strategic landscape design)were only necessary on a few areas around the building.
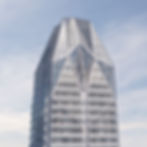
The stone wall patterns of traditional architecture of Chechnya are reinterpreted in the contemporary design of the Akhmat Tower and represented in a three dimensional developed glass, aluminum and stainless steel wall. The unitized curtain wall has a three-dimensional shape that protrudes at each floor and slopes back toward the main volume, thereby reducing glare and solar gain to the façade (see Figure 8). The slope of the wall in essence creates a horizontal sunshade which reduces glare and solar gain to the overall façade. The shingled wall has a chamfered corner, which forms a stainless-steel accent piece that catches the sunlight (see Figure 9). Operable mullions are fully integrated with the wall design, hidden within the profile of the façade mullions within each curtain wall unit, enabling the façade to have a strong consistency. Specifically designed picture windows at the corners offer enhanced views while also helping wind performance.
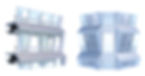
The exterior wall of Akhmat Tower is a next-generation development of the architects’ FKI Headquarters exterior wall in Seoul, which was completed in 2014 and was tilted downward 10 degrees. During the design of the FKI project, solar analysis showed that the greater the wall’s downward tilt, the less glare and solar heat gain would be realized versus a flat curtain wall. The typical Akhmat Tower wall has a 14-degree downward tilt, which was demonstrated by solar radiation analysis to have reduced solar gain, as compared to a lesser tilt.
The incorporation of the 80-millimeter-tall rotating ventilated mullions was driven by a programmatic need for natural ventilation up to nearly 400 meters in height for the residential units. On windy days, especially on the upper floors of supertall buildings, traditional operable windows tend to be used less frequently by occupants, due to powerful wind gusts flowing through the occupied floors, and difficulty opening or closing the windows. The operable mullion provides controlled natural ventilation, utilizing a button to open and close the device. This encourages natural ventilation to be used for more of the year, and alleviates some of the perceived safety concerns associated with open windows at such heights. Since the benefits of the operable mullions (less energy spent on air conditioning) are primarily dependent upon occupant behavior, further study will be required once the tower is built, in order to determine more definitively what the actual savings will be, and how the operation of the mullions will relate to the stack effect within the tower. A critical consideration for the manufacturers of this curtain wall is meeting or exceeding specified air-infiltration standards. In the future, the ability to link the operable mullions to the Building Management System (BMS) could provide valuable data and further optimize their use.
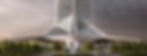
The texture and tilt of the exterior wall is continuous from the bottom of the tower pinnacle to the canopies at Level 2. As the wall reaches the bottom of the tower, it flares dramatically at the base, forming the eight-story atria that frame each lobby of the building (see Figure 10). The atria create a double-skinned wall – a monumental volumetric space that can be viewed from the lobbies below and from the lower office floors (see Figure 11).
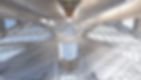
The atrium base extends out to form glass wings that act as sky-lit spaces, which frame the entries to the lobbies. Triangular, shingled canopies extend dramatically over each drop-off. Operable metal louvers within the atrium exterior wall system are linked to the smoke evacuation system, using the tapering shape of the atrium to help draw air upward, and out (see Figure 12).

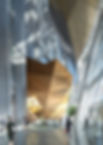
The form of the tower base informs the unique spatial opportunities of the interior design of the office, apartment, and hotel lobbies, and became a primary influencer of the design. The office and apartment drop-off elevation is situated below the hotel lobby on Level 1, allowing for a continuous space for the hotel program above, with an almost totally circular flow for occupants around the core at Level 3. Both upper and lower lobbies take advantage of the soaring, naturally lit, and sculptural spaces created by the tower base form outside of the tower columns (see Figure 13). The structure creates a sense of a screen to the office and apartments’ inner lobbies, respectively, which naturally form more intimate spaces. Exaggerated column enclosures allow passive air to circulate by way of a series of integrated grilles and ducts that move the air though natural convection. The tower base form and structure create a virtually column-free space for a 700-square-meter ballroom that has column-free and sunlit prefunction area (see Figure 13).